Selective formation of cross-catenanes - pairs of different interlocking molecular rings
The audience watches spellbound as the magician on stage pushes solid-looking rings together to form a chain, pulls them apart, and then links them again. Chemists have also mastered this trick: a system of two or more molecular rings that are interlinked but not chemically bound to each other is called a catenane (from Lat. catena = chain).
Japanese researchers working with Makoto Fujita at the University of Tokyo used two different cyclic palladium complexes as "magic rings". The palladium atom acts as a kind of "clasp" that closes the two ends of a long ligand into a ring. The first complex is a simple, flat ring. In solution, these rings immediately form double catenanes (linked pairs). The second complex also has two small side chains that stick out of opposite sides of the ring like poles. This type of ring is significantly more reserved; it takes four to five hours for all of the molecules to partner up in solution. Why the difference? The answer lies in the reaction pathway. In order for two rings to link up, the clasp of one of the rings must open so that it can thread itself through the other ring and close up again. The threading process is no problem for the simple rings and thus is fast. For the other type of ring, the side chains hinder the threading, thus making it slower. So why do these rings link at all? Interactions between components of the two rings make the catenated form of the molecules substantially more energetically favorable than individual rings tumbling in solution. If the two types of ring are mixed in a 1:1 ratio, they exclusively and without hesitation form only the mixed pairs (cross-catenation). Why? In the time it takes the inactive rings with the poles to even begin threading, the agile smooth rings have already threaded and unthreaded themselves several times. It makes no difference to them if their partner is of the same or a different type. However, only the exclusive formation of cross-catenanes allows the whole system to quickly reach the desired energetically favorable state, in which all rings are paired. In the long term, only freezing preserves all of the cross-catenanes; at room temperature pairs of matching rings eventually also start to form. After about a week, an equilibrium between the three possible types of catenane, in a purely statistical 2:1:1, distribution is established.
The formation of molecular superstructures by a self-organization process can, as the cross-catenation described here demonstrates, be strongly dependent on the speed of individual reaction steps, thus making it a highly promising, but so far little used, method for obtaining desired structures.
Most read news
Other news from the department science
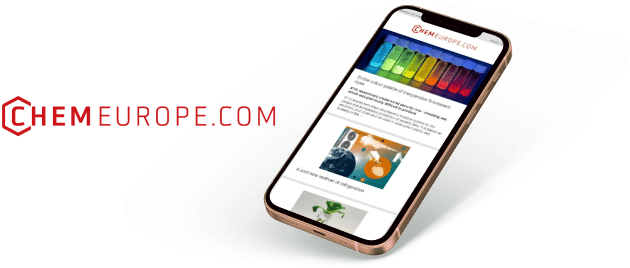
Get the chemical industry in your inbox
From now on, don't miss a thing: Our newsletter for the chemical industry, analytics, lab technology and process engineering brings you up to date every Tuesday and Thursday. The latest industry news, product highlights and innovations - compact and easy to understand in your inbox. Researched by us so you don't have to.