'Molecular movie' captures chemical reaction on atomic scale
A team of physicists from the University of Nebraska-Lincoln, Stanford University and Europe has captured the clearest glimpse yet of a Photochemical Reaction - the type of light-fueled molecular transformations responsible for photosynthesis, vision and the ozone layer.
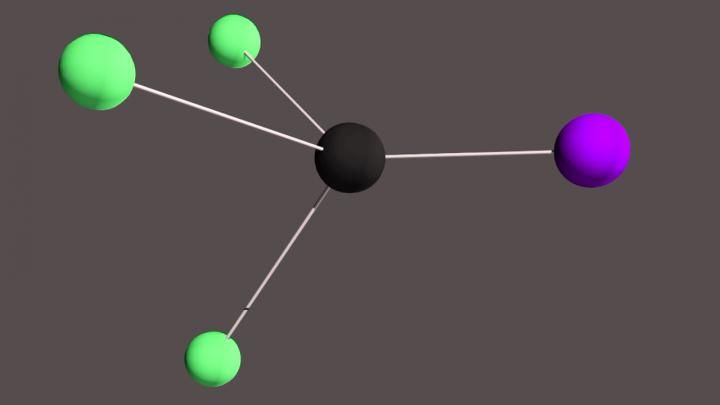
A rendering of the five-atom molecule -- featuring three fluorine atoms (green), a carbon (black) and an iodine (purple) -- whose dynamics were captured by new research published in the journal Science. By striking the molecule with a laser and recording the way that pulses of electrons bounced off it, the researchers managed to track the motion of its atoms and how the iodine breaks free of its bond with the carbon. Such detailed insights could eventually inform the design of molecules better at storing energy or kick-starting other chemical reactions.
Stanford University / SLAC National Accelerator Laboratory
The researchers precisely recorded how the atomic nuclei and chemical bonds of a five-atom molecule responded when being struck by a laser. The team's study marks the culmination of a years-long effort to advance the quality of "molecular movies" from that of a rudimentary stop-motion animation to a high-definition motion picture.
"People have been modeling these things for a long time, but there was really no good way to test if these models were correct," said Nebraska's Martin Centurion, a Susan J. Rosowski Associate Professor of physics and astronomy. "There is now a way to compare, in detail, what is happening experimentally with what our models would predict.
"The reason that's very important is because there are many, many different molecules, and you can excite them with many, many different wavelengths (of light). There's no way you could ever do experiments on everything, so at some point, you need some sort of model that's reliable."
Though refining those models will demand years of work and many studies, Centurion said, the Science study has established a new benchmark for using experimental data to better understand photochemical reactions on the atomic scale.
That comprehension could eventually yield important applications, he said, whether in the form of solar cells that can store the energy they collect or custom molecules better at catalyzing the reactions that can produce renewable fuels.
Nuclear family
The bonded atoms that form molecules get an energy boost when struck by photons, the fundamental components of light. That energy can have many effects: kick-starting the atoms into temporary motion; rearranging them into new structures; even snapping their bonds to form other, smaller molecules.
Since 2012, Centurion and colleagues at Stanford's SLAC National Accelerator Laboratory have worked toward capturing these atomic dynamics by striking gas-suspended molecules with a laser and pulses of electrons traveling at nearly the speed of light.
The patterns that those electrons form after bouncing off the laser-excited molecules provide information about the location of their atoms. By continuously recording those locations, then stitching them together much like the frames of a motion picture, the team previously managed to track the dynamics of simple molecules: carbon disulfide, nitrogen, iodine.
This time around, the researchers recorded the behavior of a more complex molecule - trifluoromethyl iodide - featuring three fluorine atoms, one carbon and one iodine. In doing so, they captured the molecule's iodine atom breaking free of its bond with a carbon atom: a photochemical reaction.
The team also discovered that the molecule can absorb either one or two photons. Like all molecules, trifluoromethyl iodide randomly rotates when floating in a gas. Centurion and his colleagues found that molecules parallel to the electric field of approaching light have no chance of snagging two photons but their highest possible chance of absorbing one. The reverse is also true: Molecules perpendicular to the electric field cannot receive just one photon but have a good chance of absorbing two.
Both phenomena can break the molecule's carbon-iodine bond, the study found, but do so in different ways. The one-photon reaction is much simpler, Centurion said. When the molecule absorbs a single photon, the electron orbiting the iodine nucleus quickly jumps to a higher energy state, causing the iodine to spring away from its neighboring carbon atom and strain their bond to the breaking point. The large mass of the newly liberated iodine atom means that it remains close and strongly repels the lighter carbon atom, which stays tethered to the three surrounding fluorine atoms but begins sling-shotting back and forth before eventually coming to rest.
By contrast, the complexity of the two-photon reaction led the team to plot branching, Chutes and Ladders-esque graphs that detail how the distribution of the molecule's newfound energy can affect the movement of its atoms and the springing action of its carbon-iodine bond. All of those factors, and others, determine the overall likelihood and timescale of the photochemical reaction.
But the precision of Newtonian physics gets replaced by the probabilistic laws of quantum mechanics at the atomic scale, where the position and momentum of an atomic nucleus or its orbiting electron can have several potential values, each with some likelihood of being observed at a given moment. And the actual outcome of one variable can then affect the probabilities of another, which can modify those of yet another, and so on, resulting in many potential paths to a few endpoints.
Some of those paths lead to the iodine atom immediately breaking free; others have it springing back and forth one or multiple times before separating from its carbon neighbor. Despite that complexity, the team managed to identify which values of the molecule's variables - including the energy states of electrons orbiting each atomic nucleus and the relative positions of those atoms - chart the most direct path to a photochemical reaction.
"It's sort of a unique point in the energy landscape, because it's ... what drives a lot of these transitions in molecules from one state to another, and it can drive these transitions very fast and very efficiently," Centurion said. "Sometimes, you have a molecule, and then you put energy in it, and in principle, there are many ways that this energy can be distributed.
"This helps explain why the molecule, when you pump light into it, goes from A to B instead of going to C or D or all of these other possible paths."
To react or not to react
Technical improvements at the SLAC laboratory have steadily allowed the team to shorten the duration of the electron pulses that it fires at molecules, enabling it to capture more frames of molecular motion. Whereas the team previously fired pulses lasting 200 femtoseconds, it recently reduced that to roughly 150 femtoseconds - a timespan that compares to one second as one second compares to about 211,000 years.
Centurion said the team will soon use the improved set-up to examine how DNA bases - the fundamental components of genetic code - react to ultraviolet light. DNA somehow transfers most of UV light's energy into heat rather than channeling it into a photochemical reaction, which would damage it. Learning more about how could inform the design of molecules with a similar resistance to unwanted reactions.
The opposite would also prove useful, he said.
"If you want to build solar cells, you want a molecule that converts all the energy into some sort of chemical reaction that you can use," Centurion said. "In the ideal case, you would have something that very efficiently absorbs the energy and then just stores it. Right now, a solar panel doesn't help you at night. But imagine you have (a material) that absorbs most the energy and then just stores it right there, maybe in a different chemical structure. Then you give it a little trigger, and it releases the energy slowly. Storage is becoming more and more of an issue, because nobody wants to have a huge battery in their basement."
The lessons learned by recording the photochemical reactions of increasingly complex molecules might also help engineer better catalysts - molecules that can themselves trigger and accelerate reactions, such as the splitting of water into oxygen and hydrogen. The same molecule might even catalyze different reactions, Centurion said, depending on the wavelength of light that strikes it.
"When they're whole, they're non-reactive, and then whey they break up, they can trigger some chemical reactions," he said. "We are starting to look at some of these molecules in the gas phase, and the idea is just to understand the fragmentation process. If you hit it with this frequency, what fragments do you make? If you hit it with this other frequency, what other fragments will you make? How fast will they come out?"
Research teams across the country are now signing up to use the SLAC lab's electron camera and produce their own molecular movies, Centurion said, each of which could reveal the story behind a different photochemical reaction.
"That's good, because different people have different interests," he said. "I have some ideas, but other people have different and better ideas. So rather than us trying to come up with all of the interesting examples, I think the best way is to open it up so other people can use it."